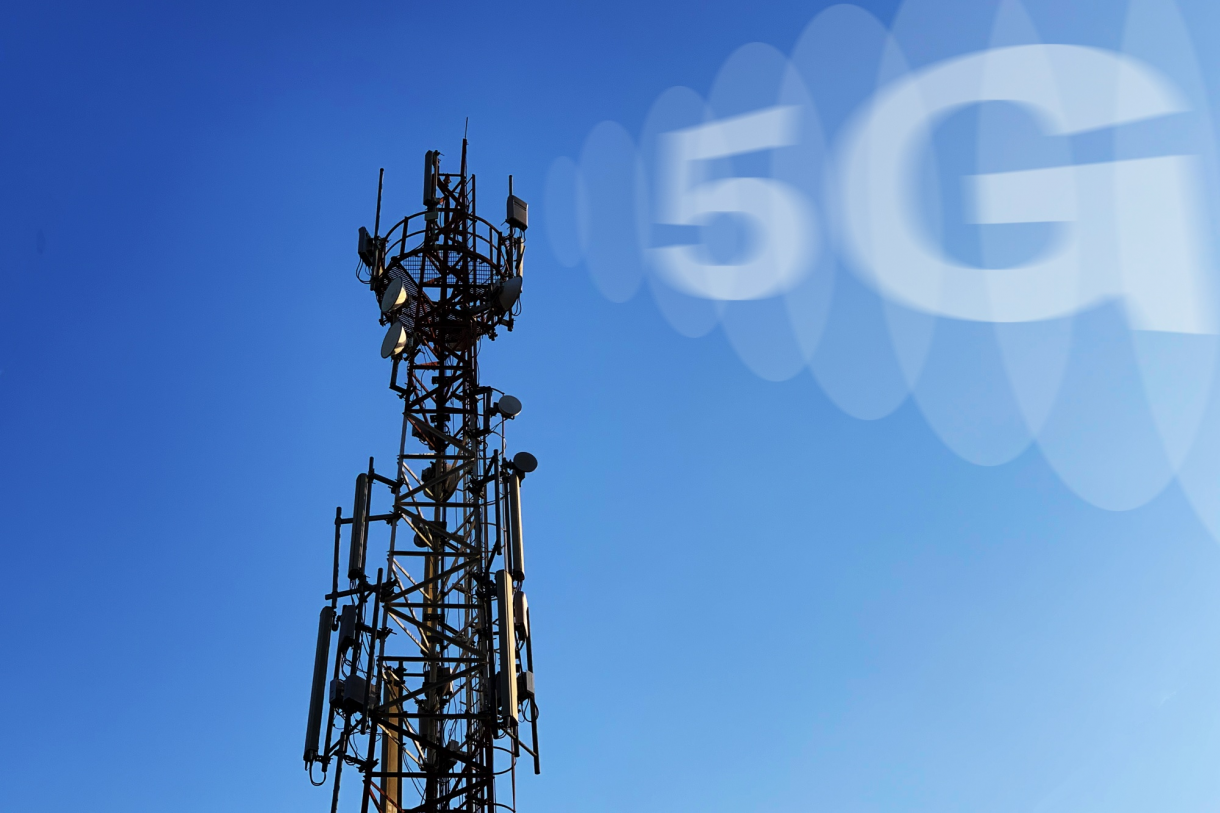
Share this Post
Why does 5G network technology matter?
The fifth-generation cellular network technology, 5G, is about to accelerate the overall pace of our daily lives and become a key part of our critical digital infrastructure. By enabling greater data rates, increasing machine-to-machine communication as well as lower latency, it is the crucial enabler for optimizing existing manufacturing processes and digital services as well as supporting emerging innovations such as autonomous driving or smart cities. Consequently, 5G offers great potential for economic growth. At the same time, it also entails risks on issues such as national security, individual privacy and economic dependence.
In light of the starting network roll-out, 5G is positioned at the heart of the great power contest for technological leadership between the US (United States) and China (the People’s Republic of China). The core of the conflict boils down to the question of whether government-linked Chinese telecom companies should be allowed to supply key components for the 5G architecture. This close relationship between vendors such as Huawei or ZTE and the Chinese Communist Party (CCP) raises concerns in terms of national security and data privacy as well as potential loss of its economic and technological edge and the lack of a level playing field. As a result, the US banned and sanctioned these Chinese companies and has pressured other countries to follow suit. Countries around the world face this multidimensional and intricate challenge where political, economic and technical parameters are mutually dependent.
This backgrounder sheds light on the significance of 5G and its technical, economic and political context. First, it outlines the social-technical context as well as the basic technical, economic and political parameters surrounding 5G. Second, it describes the main actors involved and their positions towards the main 5G issues. Third, the latest developments and upcoming trends will be outlined.
The historical and socio-technical context of 5G
Over the course of the current decade, the global roll-out of 5G networks will take place, facilitating the upscaling of existing services and the emergence of novel innovations. The first 5G networks were launched in 2019.[i] However, the overall trajectory of cellular networks goes back more than 40 years.
Simply put, 5G is the ‘fifth generation’ of wireless network technology (or mobile communications). The evolution started with 1G in the late 1970s and during the 1980s. Wireless transmission was based on analog radio signals,[ii] thus offering only voice calls and leading to the advent of the first (and very expensive and bulky) mobile phones with limited battery power. As analog signals are very prone to disturbance from external sources and unencrypted, communication was not secure and of poor sound quality; the high rate of dropped calls posed a further challenge. 1G also had limited coverage and capacity. Additionally, roaming between network operators was non-existent.
With the introduction of 2G (also known as Global System for Mobile (GMS) standards) in the early 1990s, major weak points of the preceding generation were remedied. The switch from analog to digital signaling enabled the more effective encryption of mobile conversations and the transfer of data services, such as SMS (Short Message Service) and MMS (Multimedia Message Service). Consumer adoption of mobile phones significantly increased and roaming between different network operators became possible in principle.
At the turn of the 21th century, 3G (also known as Universal Mobile Telecommunications System (UMTS))[iii] facilitated mobile access to the Internet as well as email and video conferencing/streaming services, paving the way for the development and sale of smartphones. Standardized according to the International Mobile Telecommunications-2000 ITU (IMT-2000) standard, the third mobile generation allowed data rates of 384 Kbit up to 7,2Mbit/s. ‘True mobile broadband’ or the ‘Internet of applications’ was pioneered by the introduction of 4G in the very late 2000s. With higher data rates and download speed, video conferencing, streaming capabilities, Voice over IP/IP telephony or online gaming began to unfold with higher quality and higher definition.
The latest development, 5G, holds the promise of prompting another leap in wireless network technology. With its first installations at the end of the 2010s, the advances in data transfer rates, download speed rates (4G: 300 up to 1000 MBit/s vs. 5G: 10,000 MBit/s under ideal conditions), providing lower latency (4G: 50 milliseconds vs. 5G: 1 millisecond) and operating a wide range of devices are about to heavily support the Internet of Things (IoT) and future applications with major implications for economic and security competitiveness. By potentially enabling several novel innovations at the societal and industrial levels, and a stronger transition from handset-to-handset (e.g., phone-to-phone) to more extensive machine-to-machine communication, 5G differs from its precedent generations (see Annex 1: 5G-supported use cases). In its initial years of network deployment, however, 5G will most probably not be able to fully implement all of the desired innovations to the full extent and time will tell whether even the subsequent generation, 6G, is required for some of these.
One very important aspect of the design and deployment of cellular networks are interoperable technical specifications and industry standards, on which mobile communication systems, equipment or devices rely. In previous cellular generations, different companies and countries set different standards, meaning that certain mobile technology components could not be used in all networks or countries. Common globally agreed standards are clearly preferable, as they have profitable advantages, including quicker market launchers of equipment and the selling of components on a global scale.
Unified global standards have been the case in the 5G standard-setting process, which is mainly driven by the 3rd Generation Partnership Project (3GPP). Starting with developing of the 3G UMTS standard, the 3GPP has evolved into the prime mobile telecommunications standard body in the last two decades. It is currently guided by a consortium of seven telecommunication standards organizations[iv] from China, Europe, India, Japan, South Korea and USA, totaling 700 members (2021), among them government agencies, tech companies (e.g., Intel, Qualcomm, Fujitsu) as well as telecom vendors (e.g., Huawei, Ericsson, ZTE, Nokia) and operators (e.g., AT&T, China Mobile, Deutsche Telekom).
The main output of the 3GPP is the publication of ‘Releases,’[v] which are packages of standardization documents. The first two of three packages of Release 15 in December 2017 and respectively in September 2018 stipulate the technical foundation for non-standalone 5G (NSA 5G) and subsequently full standalone 5G (SA 5G) (see ‘Technical parameters,’ below). These technical specifications and standards have to meet certain requirements and a key performance index (IMT-2020) generated by the International Telecommunications Union (ITU), a specialized agency of the United Nations.[vi] Consequently, the 3GPP submitted its 5G standard proposals to the ITU. After evaluating the 3GPP 5G standard proposals, the ITU formally endorsed them in July 2020 as the International Mobile Telecommunications-2020 5G (IMT-2020 5G) global standard. The IMT-2020 will be most probably extended by new upcoming 3GPP 5G Releases.[vii] Moreover, several companies and research institutes have already embarked on developing the foundation for 6G, whose first technical standards are expected in the second half of the decade, to be followed by market readiness from 2030 hence.
Basic technical, economic and political parameters: essential facts and figures
In this section, the basic technical, economic and political parameters will be displayed. It is important to note that these parameters mutually influence each other, creating a complex situation for political decision-makers.
Technical parameters
Technically, the new 5G-related functions rely on 5G’s novel network structure, which includes the network ‘slicing’ and virtualization of 5G networks, the difference between non-standalone 5G (NSA 5G) and standalone 5G (SA 5G) as well as the interplay between radio access network (RAN) and core network (Core).
Network slicing and virtualization coordinate between applications, devices or users that have very different requirements in terms of transmission rates, and latency / availability / maximum device density. The basic idea is to ‘slice’ the physical network architecture in several independent networks that are configured to effectively meet different requirements of various use cases as needed. With Software-Defined Networking (SDN) and Network Functions Virtualization (NFV), the multiple individual functions of a single hardware unit are integrated on a uniform, virtualized platform and controlled by software. On that basis, the latter creates virtual network slices providing specific functions and features for different applications.
The 3GPP standards have codified three broad sets of specifications that can be potentially applied into individual network slices to serve certain requirements. First, Enhanced Mobile Broadband (eMBB) provides higher data rates which should support, for instance, virtual reality (VR) or augmented reality (AR) services and in general higher download and upload speed rates. Second, Ultra Reliable and Low-Latency Communication (uRLLC) is designed for very time-critical applications that require very short latency times and very few or almost no gaps in communication. Thus, potential use cases include autonomous driving with its car-to-car and car-to-infrastructure communication. Third, Massive Machine Type Communications (mMTC) pave the way for advanced machine-to-machine communication and Internet of Things (IoT) applications since they should potentially allow the connection of billions of sensors and devices per unit area. However, all three functions can technically be used at the same time to a certain degree, especially with the growing roll-out of SA 5G (see below).
Another feature of 5G concerns the specific interplay between the radio access network (RAN) and the core network (Core), which are, roughly speaking, the two main components of cellular network architecture.[viii] The first is the link between the end-user mobile devices and the core network. It consists of radio base stations with large antennas that cover specific geographical areas depending on their capacity. Here, 5G RAN can be implement in different variations, including O-RAN, V-RAN and C-RAN (see Annex 2: O-RAN, C-RAN, V-RAN). In the case of making or receiving a phone call or connecting to a remote server, the antenna transmits and receives signals (radio waves) to and from the devices. At the RAN base stations, the signals are digitalized and transferred to the core network owned by the telecom company, where the traffic (voice, data, video traffic) of the end users are aggregated and further processed within centralized data centers.[ix] This includes the management of routing and the process of charging phone calls and data use by telecom operators as well as provision of internet access to the users.
However, with the introduction of 5G, the task division between RAN and Core – in contrast to the preceding generations of mobile network technologies – are no longer clearly separable. To provide some of the new 5G-enabled services, previous Core functions have to be deployed closer to the ‘edge’ of the network or, in other words, already integrated with the RAN network, for instance in the form of smaller data centers co-located with the base stations. This shortens the distances for data travel between the end user devices and the data processing infrastructure, enabling low-latency services in the context of IoT applications.
The distinction between non-standalone 5G (NSA 5G) and standalone 5G (SA 5G) refers to two sub-categories of 5G networks. In the initial phase of the 5G roll-out, non-standalone networks will be deployed by leveraging existing 4G core networks and connected them with 5G RAN to support first and foremost eMBB functions. Later on, with the installation of SA 5G, uRLLC and mMTC services as well as network slicing will be implemented more frequently, which will require new infrastructure, including 5G core networks. Even though the first SA 5G installations were launched in 2021, a full shift towards SA networks will only take place in the course of the decade, with large variations depending on the region.
Last but not least, 5G has specific wireless spectrum frequency bands. Wireless spectrum refers to a range of radio waves that transmit information wirelessly from user equipment to cellular base stations. These radio waves are measured by frequency, namely the number of waves that pass a fixed place per second. 5G comprises the low-band (approx. 600–900 MHz), mid-band (2.3–4.7 GHz) as well as high band (>24 GHz) frequencies. These frequency bands roughly reflect the range and intended use of 5G capabilities. In general, higher frequencies provide faster services, but have shorter ranges for radio waves.[x] The generation and distribution of wireless spectrum are regulated by national laws, for instance through spectrum auctions, and by international bodies such as the ITU.
Economic parameters
The economic parameters involve the (potential) economic impact of 5G and its importance for economic competitiveness, the 5G innovation ecosystem and the global players and market share in the context of RAN.
While the overall network roll-out and the market readiness of 5G-enabled innovations are still in early stages, different estimates assume that 5G-powered services and applications in several sectors will add US$1.3 trillion to global GDP by 2030 or US$2.3 trillion by 2035. The sectors that benefit most include manufacturing (industrial IOT and automation), healthcare, public services, finance and insurance, media and consumer applications (e.g., smartphones, AR/VR headset) or transportation (e.g., autonomous driving).
Thus, depending on the definition, the 5G ecosystem encompasses several (upcoming / upgraded) devices such as smartphones and tablets, innovations such as smart cities, industrial IoT and autonomous driving as well as network components (e.g., RAN, core networks). Relevant players in the evolving 5G core network market players are, among others, Huawei, Cisco, Nokia, Hewlett Packard, Samsung, Ericsson, NEC.
Market power is also reflected in the possession of standard essential patents (SEP). To implement the 3GPP cellular standards, a great number of patented technologies and innovations (e.g., cellular devices such as 5G-compatible smartphones and network components) are needed or are perceived as ‘essential.’ The individual parts or building blocks of these products are then protected by SEPs. Companies that own patents that are essential to the standard can license the SEPs on fair, reasonable, and non-discriminatory terms. In other words, if companies want to construct 5G components according to the standards with the necessary patented technologies, they have to pay royalties to the patent holder.[xi] According to analyses of IP analytics, Huawei (China) (15.39%) has so far declared the highest number of 5G-relevent patents, followed by Qualcomm (USA) (11.24%), ZTE (China) (9.81%), Samsung (South Korea) (9.67%) and Nokia (Finland) (9.01%).There is a presumed overall tendency whereby some companies are engaged in ‘over-declaration’ of SEPs, signaling its presumably high market competitiveness; however, the exact numbers are unknown, as not all data concerning SEPs are disclosed to the public. Thus, by applying essentiality rates,[xii] the picture changes with Samsung (18.52%) in the lead, followed by Nokia (11.44%), Qualcomm (10.75%) and Huawei (8.38%) (all as of February 2021).[xiii]
The political debate (see ‘Political parameters,’ below) centers on ‘traditional’ RAN due to the special interplay of its specific global market concentration and its implications for national security. There are only a few telecom vendors among whom the entire global market is divided. In 2020, the global RAN market was distributed as follows: Huawei (31%), Ericsson and Nokia (Finland) (both 15 %), ZTE (10%), Samsung (2%). This distribution, so far, has remained virtually unchanged in 2021. Due to heavy investments and developing SEPs in recent years, it can be expected that Samsung, in particular, will gradually expand its global share over the coming decade. The RAN components (antennas, RRU, BBU, etc.) supplied by these five companies consist mainly of closed vendor-proprietary hardware and non-interoperable software. This leads to lock-in effects, meaning that individual RAN components are not compatible with products from other vendors, giving rise to the need for long-term commitments to one specific company.
Interestingly, the global 5G RAN market setting is unique in contrast to other major fields of technological competitiveness: Whereas the Chinese telecom giants Huawei and ZTE are among the leaders in RAN technology, the US as ‘technological superpower’ is lacking these companies. The absence of homegrown industrial leaders is rooted in a set of US laws and policies that can be traced back to the mid-1990s, which has led to the foreign takeover of US telecom players. Previous US administration and private sector actors did not foresee the strategic significance of industrial capacities insofar as cellular network technology was concerned. Europe, which in recent decades has lost ground in other innovation fields such as cloud computing and semiconductors, is well positioned globally with Ericson and Nokia.
However, through the potential introduction of alternatives to traditional RAN such as Open-RAN, C-RAN or V-RAN, there are upcoming opportunities for new players to enter the market and a potential breakup of the oligopolistic structure. It is estimated that the global Open-RAN market will account for more than 10% of the overall RAN market by 2025, starting from 2% in 2021. Also, the V-RAN market is poised to grow, from US$ 1.1 billion in 2020 to US$ 6.4 billion by 2030, as well as the C-RAN market with the rise at a CAGR of 10.4% from 2019 to 2026
Political parameters
Technological innovations are increasingly becoming one of the key foundations for economic, political and military competitiveness. As a logical consequence, the great power contest between the US and China is increasingly defined by the ability to innovate and possess key technologies. Besides AI and semiconductors, 5G technology has been at the core of the technology-based rivalry between both actors in recent years. In particular, the combination of the technical and economic parameters coupled with the geopolitical power game creates an intricate situation not only for the USA and China, but also for almost all countries around the world planning to build 5G infrastructures. Ultimately, however, it boils down to the decision whether state-connected Chinese companies should be allowed to provide equipment to countries’ 5G critical infrastructure.
The controversy around the market power and first-mover actions of Huawei and ZTE is framed by China’s overall quest for technological advances to upgrade its economic, political and military prowess. The Chinese Communist Party (CCP) has launched numerous strategic initiatives aiming at strengthening the technological capabilities of China. Examples include “Made in China 2025” to enhance Chinese industrial and tech fields such as 5G and related sectors such as IoT, AI or semiconductors; the ‘Digital Silk Road’ (DSR), which is the ICT constituent of the large-scale ‘Belt and Road Initiative’ (BRI) and seeks to provide overall connectivity with 5G networks, terrestrial and submarine cables, data centers or its global satellite system BeiDou to several world regions; and the 15-year blueprint ‘China Standards 2035’ which aims to enhance the clout of Chinese actors in international standardization bodies and to shape standards globally.
Tech giants such as Huawei and ZTE assume an important role in these contexts. It still remains questionable how close the links are between these companies and the CCP. Nevertheless, it cannot be denied that the strategic global positioning and innovation power of the two Chinese companies in the realm of 5G are rooted in state-driven efforts. As early as 2013, the IMT-2020 5G Promotion Group was established by the Ministry of Industry and Information Technology (MIIT), the National Development and Reform Commission (NDRC) and the Ministry of Science and Technology (MOST), an alliance comprising, among others, research institutes (e.g., China Academy of Information and Communications Technology (CAICT)), telecom operators (e.g., China Mobile, China Telcom) and vendors such as Huawei and ZTE. While China was sidelined during 3G and had a limited role in 4G global standard-setting, this broad alliance helped to strategically bundle and align successful Chinese 5G-related contributions to 3GPP, benefitting homegrown companies.
The increasing intertwined relationship between the private and public sector, in particular under President Xi Jinping, is also reflected in the large amount of state-subsidies, financial assistance and tax exemptions that Huawei and ZTE have reportedly been receiving from the Chinese state, skewing the level playing field with its global competitors to some degree. This rather opaque governance structure of these companies contributes to the picture of heavy-handed government involvement. The Chinese state also exerts influence on these companies by imposing strict regulations as well as demanding they set-up Chinese Communist Party (CCP) branches within them. Huawei and ZTE have repeatedly assured their independent nature.
The blurred lines between the private sector and the CCP as well as vague domestic laws lie at the core of the national security concerns with regard to 5G equipment provided by Chinese telecom companies. It comes down to the potential risk that the Chinese state will co-opt the telecom companies and conduct cyber espionage or even shut down the networks (‘kill switch’) in the event of a conflict with the help of the provided 5G equipment.
Technically, there are several concerns that have been voiced by intelligence services and political decision-makers that Huawei and ZTE will install hidden ‘backdoors’ in their 5G products to remotely access the networks it helped constructed. Government and media reports have repeatedly accused Huawei and ZTE for being previously engaged in cyber espionage. For instance, US government officials have reportedly claimed that Huawei has made use of backdoors for malicious activities across its 4G equipment since 2009. During a hearing in the Senate Intelligence Committee in 2018, six US intelligence chiefs – including the heads of the CIA, FBI and NSA – warned against using Huawei and ZTE services. In 2019, Polish prosecutors arrested a Huawei employee on suspicion of conducting espionage activities. The UK-based Huawei Cyber Security Evaluation Centre (HCSEC), which is run by the National Cyber Security Centre and responsible for evaluating and testing equipment provided by the company, states in its latest annual report that there is “no overall improvement [by Huawei] over the course of 2020 to meet the product software engineering and cyber security quality expected by the NCSC.” There were also reports that data from the computer systems of the African Union headquarters in Addis Ababa, which are provided by Huawei, were illicitly transferred to Chinese authorities. In addition, Huawei and ZTE are facing growing international criticism for their involvement in providing AI surveillance tools (e.g., CCVT cameras with facial recognition) to repress the Uyghur minority in Xinjiang and for exporting these technologies to authoritarian governments globally.
The technical risks and attack surface for these potential malicious activities have increased and become trickier through the transition to 5G architecture. As described (see ‘Technical parameters’), a clear-cut separation between RAN and core network is no longer feasible for materializing many functionalities of 5G. Thus, it is no longer possible to simply allow a vendor into the RAN network and exclude this same provider from the core network, where the central data processing takes place. In contrast, some telecom operators have used Huawei equipment in 4G RAN networks, but opted for another vendor for the core in the 4G architecture.
Legally, Chinese intelligence laws leave much room for interpretation and might entail the forced cooperation of private companies for information gathering. For instance, the 2015 National Security Law stipulates that Chinese citizens and companies have the “responsibility and obligation to maintain national security.” More concretely, the 2017 National Intelligence Law basically compels Chinese firms to “support, assist, and co-operate with state intelligence work” and to guard the secrecy of such work. Taken together, this legal foundation might suggest a close collaboration between the CCP, its intelligence services and the private sector by necessity, even if companies such as Huawei propose the signing of ‘no-spy’ agreements or ‘trustworthiness’ declarations.
What is happening at the moment? Who are the main actors involved? What do they want? What do they do?
Given these national security concerns, one of the most pressing questions is whether or not certain vendors should be allowed to supply components for 5G architecture and under what conditions this decision should be made. As of June 2021, 58 countries had 5G networks, most of them developed countries located in Europe, North and South America as well as Southeast Asia. According to the GSMA Association, one-third of the world’s population are likely to be covered by 5G networks in 2025.
From the very beginning, the US has adopted a harsh stance towards the Chinese vendors. Already in April 2018, the Department of Commerce banned U.S. companies from exporting technology to ZTE for seven years since the Chinese company had illegally traded its products that consisted of US technologies (e.g., US-based semiconductors from Qualcomm or Android, Google’s operating system) with North Korea and Iran; after ZTE paid the requisite fines in June 2018, the ban was lifted. In May 2019, the United States Department of Commerce’s Bureau of Industry and Security (BIS) placed Huawei on the Entity List (a trade restriction list) since the US authorities classified the company as “engaged in activities that are contrary to U.S. national security or foreign policy interests.” US companies are only allowed to export, reexport, or transfer items to Huawei if they fulfill special strict license requirements. These requirements were tightened in 2020. Finally, in June 2020, the U.S. Federal Communications Commission (FCC) officially designated Huawei and ZTE as national security threats, with the result that US companies cannot use either vendor for their 5G networks.
The Trump administration has also taken its tough stance against the Chinese vendors to the international level and pressured its allies – in particular in Europe – to follow suit. Several high-ranking US officials even warned partners that Washington will limit intelligence sharing with countries using Chinese-supplied 5G components. This international stance was manifested by the Trump administration with the ‘Clean Network program,’ an initiative to persuade countries to exclude Chinese software and hardware from their networks with a focus on only allowing ‘trusted vendors’ into 5G networks; 53 countries have signed on to the program (as of December 2020). In September 2021, the Transatlantic Telecommunication Security Act (TTSA) with the aim of incentivizing investments in non-Huawei 5G equipment in Central and Eastern Europe was introduced to the US Congress.
Around the world, the debate over the admission of Chinese vendors into the national 5G networks is still very controversial and includes several trade-offs between national security concerns, economic interests, and considerations pertaining to the long-term relationship with the US and China. In Europe, different approaches to this decision have started to emerge. Thus, the EU (European Union) has been pushing for a concerted approach among its members, even though the final decision is left to the individual nation states.[xiv] In March 2019, the European Commission proposed that member states share information of 5G-related risks with each other and the ENISA (European Agency for Cybersecurity) This resulted in an EU risk assessment report released in October 2019, outlining a broad array of cybersecurity risks from 5G networks. This also includes risks relating to individual vendors stemming from outside of the EU.
This coordinated approach by the European Commission was further pursued through the release of the non-binding paper titled “Cybersecurity of 5G Networks – EU Toolbox of Risk-Mitigating Measures,” which is, strictly speaking, a how-to guide for managing 5G vendors. Without explicitly naming Huawei and ZTE, the paper (also known as the “Toolbox”) outlines the issue of potential state-led interreference via so-called ‘high risk suppliers’ and raises the possibility of limiting or even banning vendors from certain sensitive components such as the core network. Furthermore, the Toolbox encourages member states to adopt a multi-vendor strategy to avoid over-dependence on a single supplier. Besides the risk-related issues, the Toolbox also places emphasis on the necessity to further strengthen Europe’s comparatively strong market power in telecommunication technology, including in terms of the development of 6G.
Even though the decision of whether to exclude Huawei from the 5G infrastructure is still pending in several European states, different models of market and network access seem to be evolving. For instance, the Swedish Post and Telecom Authority (PTS) decided to ban Huawei from supplying 5G equipment due to national security risks, a decision that was later approved by the domestic courts. Romania and Poland have also opted for an outright ban.
The government of the United Kingdom initially intended to enable Huawei to supply 35% of the UK’s 5G equipment. However, after an about-face at the beginning in 2020, telecom operators were banned from buying Huawei 5G equipment beginning in 2021, and a mandate was issued to remove already-installed Huawei 5G components by the end of 2027. A similar phase-out and limiting approach is being taken by France, since its cybersecurity agency ANSSI declared in 2020 that it will not renew licenses for built-in 5G equipment made by Huawei after 2028. As a result, the French telecom operator Bouygues has to replace 3,000 already-acquired Huawei mobile antennas. Germany’s political debate about Huawei displays the difficulty and complexity of the decision. Initially in October 2019, two technical agencies, namely the Federal Office for Information Security (BSI) and the Federal Network Agency (BNetzA), issued a security catalogue on behalf of the German government, which ruled that the decision should be purely technical, without considering geopolitical aspects. Several members of the Bundestag, federal intelligence agencies and even ministries in the government itself opposed this approach, leading to a long deliberation process on how to deal with critical vendors. The new approved IT Security Act 2.0 includes a new regulation which specifies criteria for technical and political assessment of 5G vendors.
European countries such as Belgium, Croatia, Finland, Greece, Norway, Portugal, and Spain have not officially banned Huawei from their 5G networks, but their telecommunications firms have so far contracted Ericsson or Nokia to build their 5G networks. Other countries such as Hungary, Iceland, Ireland and Turkey are underway to use 5G equipment from Huawei.
Beyond Europe, the Australian government already decided in 2018 to impose an outright ban ZTE and Huawei from their 5G networks, followed by Japan and Taiwan which also banned both companies in principle. Canada is also about to ban Huawei from its networks. Even though there is no official ban in place in India yet, the Telecom Ministry left Huawei and ZTE out of its 5G trials announced in May 2021.
Most of the South American states will allow Huawei in their 5G networks, even though the debate has also raged in these countries. In the case of Brazil, for instance, the US has already offered Open RAN systems as an alternative to Huawei, while at the same time, Washington has also signaled that the security relationship with Brazil would suffer if the latter accepts Huawei. However, Brazilian telecom operator TIM will begin its 5G network rollout with equipment provided by Huawei and Ericsson. On the African continent, overall 5G adoption is comparatively very low and the number of African countries that have planned the concrete deployment of 5G networks is still below ten as of 2021. It can be expected that the Chinese vendors will occupy a strong market position in the African 5G market due to the overall strong presence of Huawei and ZTE in many African countries. For instance, approximately 70 percent of 4G base stations in Africa are made by Huawei.
The Chinese side has always denied the charges that it uses 5G equipment for espionage activities and protested in several cases against the banning of Huawei or ZTE components. In the cases of Sweden, Poland and Romania, Huawei has already intended to take legal steps. In 2019, China’s Ambassador to Berlin declared that his country can designate German cars as security threats if they will use 5G equipment from Chinese vendors. A similar approach was taken by China’s ambassador to Canada towards the government in Ottawa. The US sanctions have damaged the business of both Chinese vendors. Nevertheless, there are many countries that are still willing to acquire 5G equipment by Huawei and ZTE.
What are the latest developments? What happens next? How is it likely to unfold and what are the implications?
In an effort to curb the global influence of Chinese companies in the global RAN business and to compensate for the non-existence of US companies in this field, the US government is currently pushing for the development and worldwide adoption of Open RAN solutions, with the support of the UK government. Open RAN is perceived by US decision-makers as the entrance ticket back into the RAN market and as a potential bulwark against the market dominance by Huawei. The US Congress passed a $750 million program to support domestic 5G Open RAN market development over the next ten years in 2020. In May 2021, the Open RAN Policy Coalition was founded, consisting of a majority of US companies.[xv]
In addition, Germany has announced that it will be allocating €2 billion to finance Open-RAN development. Several companies such as Rakuten (Japan), Vodafone (UK), Orange (France) or Telefonica (Spain) have launched Open-RAN pilot projects. Nevertheless, there are also some critical voices, in particular from China and Europe, that doubt the performance of Open-RAN systems and are concerned that it might weaken current 5G market leaders from China and Europe and strengthen the competitive position of US-based companies. In addition, the EU, Germany[xvi] and both European leading companies Nokia and Ericsson have voiced security concerns around the concept of Open RAN and question the maturity of its architecture.
As the deployment of standalone and non-standalone modes and the initial application of 5G-enabled services are only starting to unfold, several countries, companies and research institutes have already begun to invest in 6G development. Given the early strategic approach by Chinese actors towards 5G, several countries and companies have embarked on maintaining their strengths in mobile technologies or potentially re-entering the market in critical segments with the advent of 6G (see Annex 3: 6G Development). For instance, the US and Japan have agreed to collaborate and jointly invest $4.5 billion in 6G research. The EU and some of its member states, such as Germany, have also started to invest in 6G, while Huawei has already been conducting related research activities since 2017. It can be expected that parallel to the 5G network roll-out and its ongoing political controversies, 6G will become another frontier of tech-geopolitics.
In conclusion, the network roll-out of 5G and the upcoming ‘race’ to 6G will remain the focus of debate due to the increasing importance of technologies for economic and geopolitical competitiveness. In particular, the question of whether Chinese vendors should be allowed into 5G networks and whether they pose a risk to national security will continue to fuel political controversy. Most countries around the world will have to navigate between the two superpowers’ preferences in choosing (or not choosing) the right vendors and will have to ask themselves how to balance economic, national security and political interests. It also remains to be seen how the potential exclusion of certain vendors will fuel the trend of ‘technological decoupling.’
Annex 1: 5G-supported use cases
- Autonomous Driving: Installing 5G networks on key transport routes such as main roads or highways might support autonomous driving, through ultra-low latency (low delay time for data transfer, such as between cars as well as between cars and the traffic infrastructure) and massive car-to-car communication.
- Smart Factory/Advanced Factory Automation: Thanks to 5G, machine runtimes can be optimized to enhance efficiency.
- Smart Cities: 5G-supported smart city applications might involve the optimization of real-time information on traffic conditions, public video surveillance or the monitoring of congestion, crowding, and public transit services. The support of 5G greatly increases the number of sensors that can be integrated into the urban infrastructure.
- Healthcare: The functionalities of 5G can benefit the healthcare IT market, for instance for telehealth, real-time remote monitoring, or in some circumstances, even remote surgery.
- Augmented Reality (AR) und Virtual Reality (VR): The low latency and potentially faster upload rates of 5G optimizes many innovations in the field of AR and VR.
Annex 2: O-RAN, C-RAN, V-RAN
Simply put, a RAN base station consists of the 1) antennas, which transmit and receives the radio signals from the devices; 2) the Remote Radio Unit (RRU), which performs the task of amplifying and digitizing the radio signals and is implemented in the immediate vicinity of the antennas; and 3) the Baseband Unit (BBU) at the bottom of the cell tower,[xvii] which manages and governs the whole base station and is connected to the core network via a backhaul connection.
In general, these components are proprietary, meaning that one has to buy all parts from the same vendor. However, Open Radio Access Network (Open RAN) is an alternative to these vendor-specific systems. Initiated by the O-RAN Alliance, an industry-driven group founded in February 2018 by mobile operators, it aims at creating standards to have open interfaces between the RRU and BBU components. A potential customer can then buy RAN components from different vendors to avoid getting locked into one specific provider. Replacing these locked-in systems might bring new players into the RAN market and break the current dominance of a small set of vendors (see ‘Economic parameters,’ below).
Virtual Radio Access Network (V-RAN) basically virtualizes the hardware-based BBU as a software on a generic hardware compute platform. V-RANs can be implemented on the basis of the Open RAN concept.
In a Cloud/Centralized Radio Access Network (C-RAN), the base station is split up since the BBU is moved to a centralized site in the form of a data center or a cloud service. The C-RAN solution has multiple benefits such as resource pooling, reusable infrastructure, simple network operations and management, decreased energy consumption, and CAPEX and OPEX reduction.
Annex 3: 6G Development
China
- In its 14th Five-Year Plan (2021-25), the CCP designated 6G as one the top priorities for future investment and research activities.
- Huawei already started to invest in 6G research since 2017, for instance at its research facility in Ottawa, Canada. It has also announced that it envisions launching its first 6G products by 2030.
- In joint research activities with China Unicom, ZTE is also investing in 6G.
USA
- In 2019, President Donald Trump proclaimed that he wants 6G “as soon as possible” and the Federal Communications Commission opened a high-frequencies spectrum for 6G experimental use.
- The Alliance for Telecommunications Industry Solutions (ATIS), comprising US companies such as Apple, At&T, and Qualcomm, kicked off the Next G Alliance in October 2021 to establish North American preeminence in 6G.
- In a joint effort, the USA and Japan have agreed to invest $4.5 billion into 5G (with a focus on Open-RAN) and 6G development.
Europe
- The EU, in a 25-member initiative,[xviii] has launched the Nokia-led Hexa-X project to develop 6G research.
- The European Commission intends to allocate €900 million to 6G research programs and 5G development over the budget period of 2021-2027 in the framework of a strategic European partnership for the Smart Networks and Services Joint Undertaking. In several other policy and strategy documents as well as speeches by high-ranking EU officials, the importance of Europe’s strength in 6G is emphasized.
- The German Federal Ministry of Education and Research launched the first German research initiative on 6G technology in April 2021, which provides €700 million for 6G research until 2025.
- The University of Oulu in Finland kicked off its 6G research flagship program in 2018 with a volume of approximately €250 million over eight years.
Japan
- The government in Tokyo intends to spend approximately $482 million for 6G research and development.
- Nippon Telegraph and Telephone Corporation (NTT), Intel Corporation (Intel) and Sony Corporation (Sony) are collaborating to develop 6G technology.
South Korea
- The government in Seoul has created a five-year plan to spend some $193 million on the development of core technologies for 6G and intends to launch the first 6G trials in 2026.
- The South Korean Institute of Information & Communications Technology Planning & Evaluation (IITP) and the US-based National Science Foundation (NSF) signed a Memorandum of Understanding (MoU) for joint research opportunities, including 6G.
- In its white paper “The Next Hyper-Connected Experience for All,” Samsung outlines its vision regarding new 6G services, requirements, technologies and standardization. It states that the earliest commercialization date of 6G would be 2028.
Further Reading
- Gallagher, Jill C./ DeVine, Michael E., “Fifth-Generation (5G) Telecommunications Technologies: Issues for Congress” (Congressional Research Service, January 30, 2019)
- Gorman, Lindsay “A Future Internet for Democracies: Contesting China’s Push for Dominance in 5G, 6G, and the Internet of Everything” (GMF, October 27, 2020)
- Gorman, Lindsay/ Yen, Hugo/ Simpson, David, “5G Tech Factsheet for Policymakers” (Belfer Center for Science and International Affairs, June 26, 2020)
- Sahin, Kaan/ Tatlow, Didi Kirsten, ”Berlin’s Preliminary 5G Decision. Limiting Damage and Learning Lessons” (DGAP, November 2019)
- Triolo, Paul/ Allison, Kevin/Brown, Clarise, “Eurasia Group White Paper: The Geopolitics of 5G” (Eurasia Group, November 15, 2018)
[i] It is actually a matter of dispute as to whether the first commercial 5G network was launched in South Korea or in the USA in 2019.
[ii] In other words, the spoken languages were encoded as analog radio signals.
[iii] High Speed Packet Access (HSPA) and HSPA+ are extensions of the UMTS standard and also part of the third generation.
[iv] ARIB (Association of Radio Industries and Businesses, Japan), ETSI (European Telecommunication Standards Institute), ATIS (Alliance for Telecommunications Industry Solutions, USA), TTA (Telecommunications Technology Association, South Korea), TTC (Telecommunications Technology Committee, Japan), CCSA (China Communications Standards Association, China), TSDSI (Telecommunications Standards Development Society, India).
[v] For instance, Release 8 in 2008 introduced the Long-Term Evolution (LTE) standards, which is often used as a synonym for 4G, but is actually an extension of 3G. Thus, LTE is also known as “3.9 G”.
[vi] The ITU has been developing these requirements together with research institutes since 2015.
[vii] For instance, the performance and functionalities of 4G were gradually extended by the publication of six different 3GPP releases over a timeframe of ten years. For 5G, Releases 15 and 16 have been already developed, with Releases 17 and 18 in the making.
[viii] One can also argue that a mobile network architecture comprises four major domains: The user equipment itself (e.g., mobile phone, laptop, etc.), the Radio Access Network (RAN), the Core Network and the transport network, which establishes the connection between RAN and Core.
[ix] Usually, RAN and core are connected via fiber backhauls or microwaves. This process step is also described as transport network (see endnote, above).
[x] Simply put, high frequencies can travel at faster speeds but at shorter distances. Conversely, low frequencies travel at slower speeds but can pass longer distances.
[xi] The processes of patent licensing and royalty payments occur after standard-setting and may take several years.
[xii] This means that the actual ‘essentiality’ of these SEP’s for 5G devices and networks will be assessed again separately.
[xiii] The business law firm Bird & Bird has also applied an essentiality rate to 5G-delacred SEPs (as of July 2020), and came to the conclusion that companies from the European Union (31.4%) hold the largest share of 5G patent families, followed by companies from South Korea, China, USA and Japan.
[xiv] The paragraphs concerning the EU’s approach is based on Sahin/Barker (2021): “Europe’s Capacity to Act in the Global Tech Race. Charting a Path for Europe in Times of Major Technological Disruption,” in: German Council on Foreign Relations (DGAP).
[xv] Among the members are AT&T, AWS – Amazon, Cisco, Facebook, Google, IBM, Intel, Microsoft, Oracle, Qualcomm, Verizon and non-US players such as Fujitsu, NEC, NTT, Rakuten (Japan), Samsung (South Korea), Telefónica (Spain) and Vodafone (UK).
[xvi] For instance, Arne Schönbohm, the president of the German Federal Office for Information Security (BSI), stated that the notion of “security by design has not yet been sufficiently specified in the existing open RAN, and in some cases it exhibits security risks.”
[xvii] In fact, the BBU is located in an equipment room close to the tower itself, connected to the RRU via an optical fiber.
[xviii] The project includes members from Finland, France, Germany, Greece, Hungary, Italy, Spain, Sweden, Turkey representing companies such as Ericsson, Intel, Siemens or Telefonica.
This Backgrounder is published as part of the German-Israeli Tech Policy Dialog Platform, a collaboration between the Israel Public Policy Institute (IPPI) and the Heinrich Böll Foundation.
The opinions expressed in this text are solely that of the author/s and do not necessarily reflect the views of the Israel Public Policy Institute (IPPI) and/or the Heinrich Böll Foundation.
Share this Post
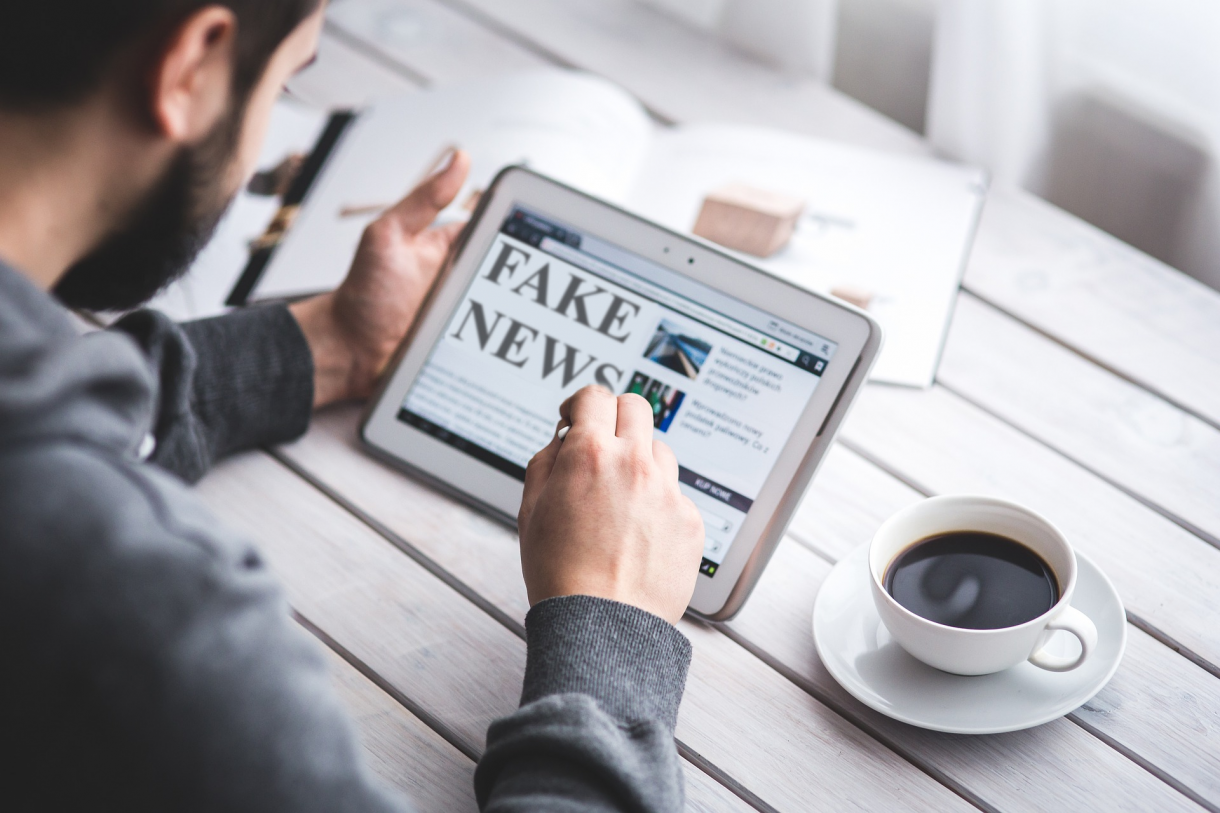
"The pandemic has put a magnifying glass on misinformation”
Disinfo Talks is an interview series with experts from around the world, who tackle the challenge of disinformation through…
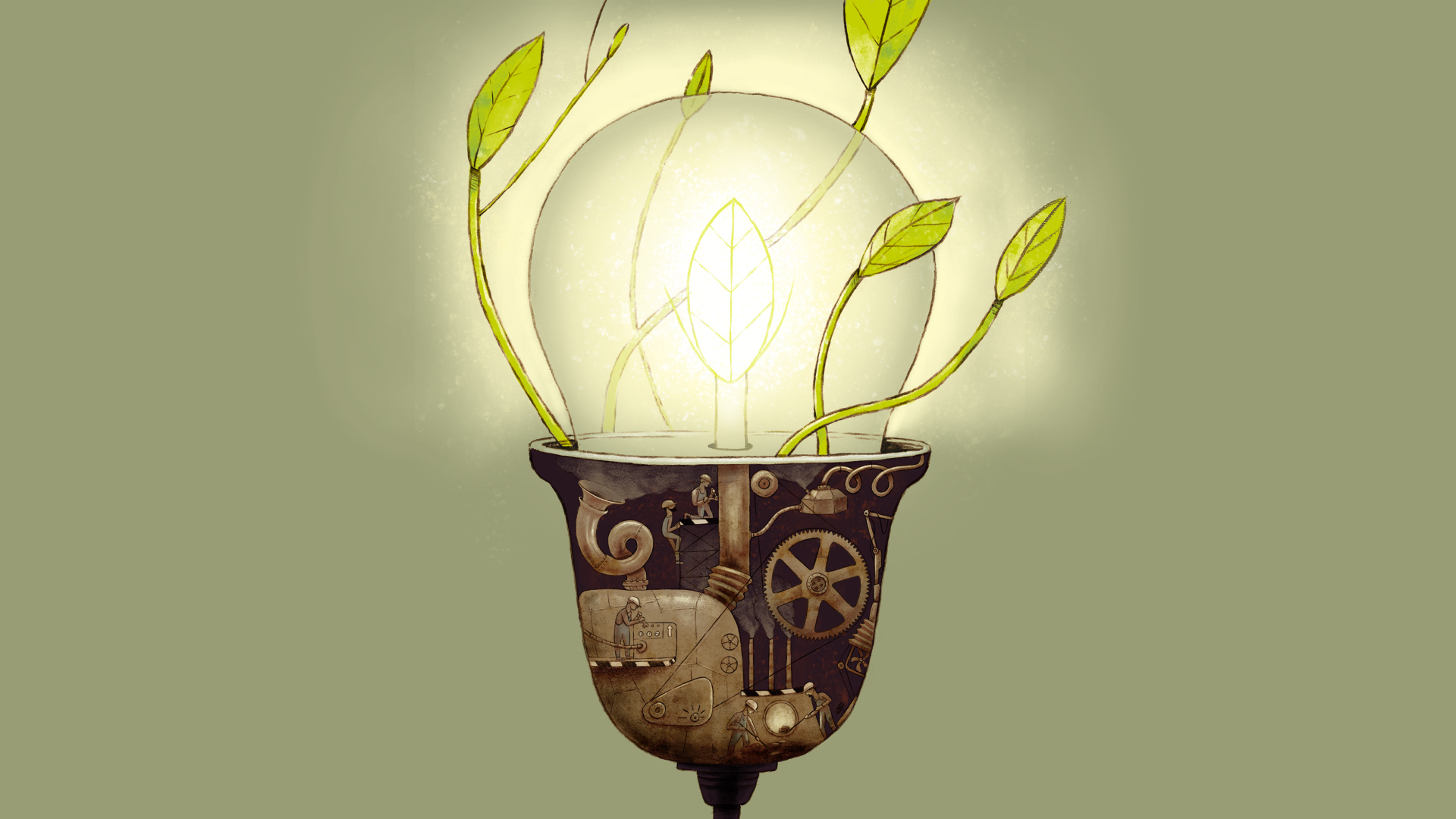
The Israeli Green Paradox: A Global Cleantech Hub Catching up with Climate Change
Edition date : October 2021 With nearly 650 companies and startups in clean technologies or Greentech (solutions that…
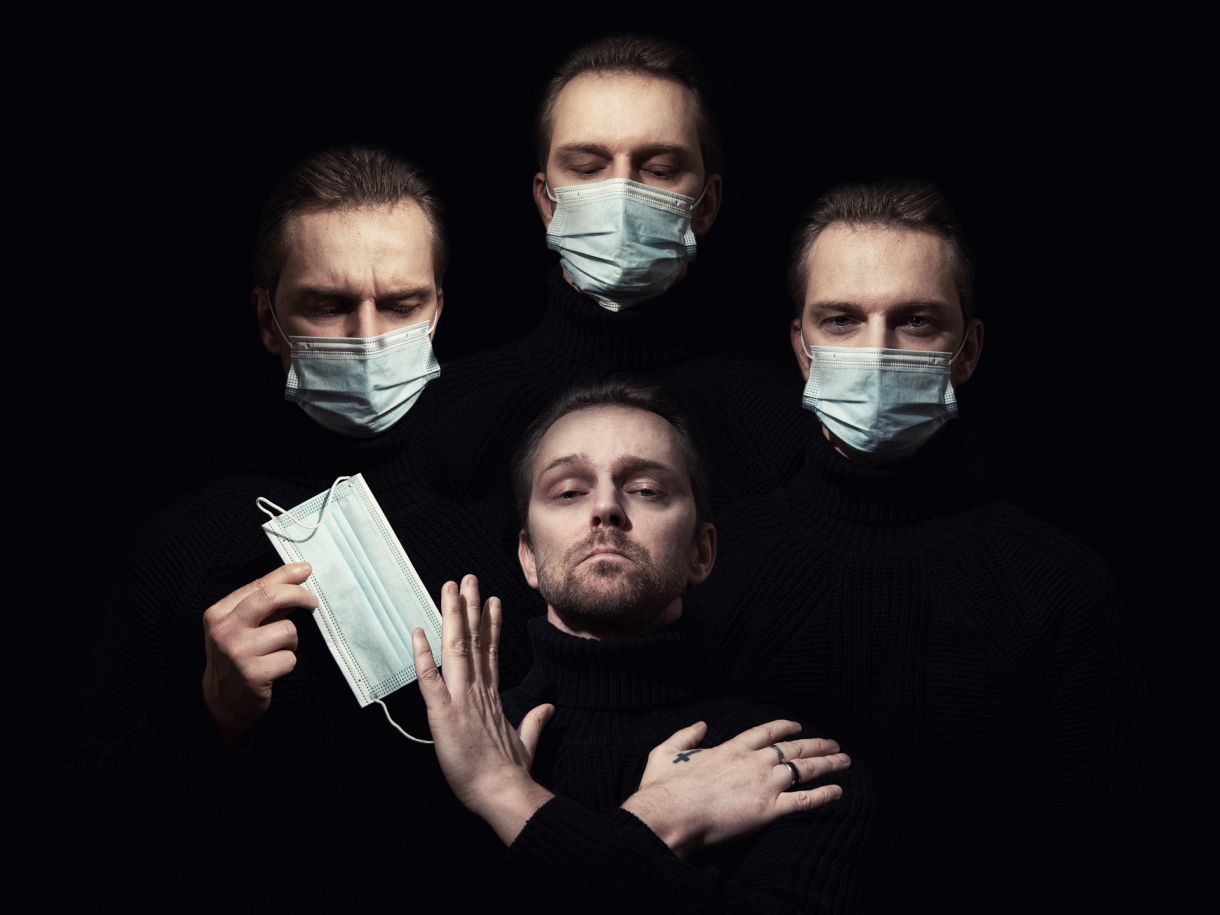
"We need to "vaccinate" people against misinformation so that they can identify suspicious information on their own"
Disinfo Talks is an interview series with experts that tackle the challenge of disinformation through different prisms. Our talks…